Development of a 3D Model of the Dermal Niche
Human skin is a complex, multilayered organ that is subjected to several mechanical and biochemical stimuli, which can modulate cell activity and affect skin function and disease. [1] In the intermediate layer of the skin or dermis, human dermal fibroblasts are embedded within a three-dimensional matrix that can generate and transmit mechanical forces. Mechanotransduction translates mechanical stimuli into intracellular biochemical signals that, in turn, drive changes in cell function. [2] Notably, mechanical stimulation, such as cyclic stretching, has demonstrated favorable effects on fibroblast proliferation and gene expression, making it useful for therapeutic applications or the production of skin substitutes. [3] Moreover, the mechanical analysis of skin elongation revealed that stretching induces changes in osmotic and hydrostatic pressure within the surrounding environment of the cell through chemomechanical coupling. [4] The effects of osmotic and hydrostatic pressure are thought to be involved in impaired wound repair, inflammation, proliferation and chromatin arrangement, although the underlying mechanotransduction mechanisms remain unclear. [5], [6] To investigate these, two-dimensional cell culture has been widely used; however, it has been recently questioned whether 2D can preserve or replicate the in vivo conditions in which cells are naturally embedded. Indeed, this can cause cells to behave far from how they would behave in the in vivo dermis, which is a soft 3D matrix. [7] Moreover, 2D systems cannot replicate the chemomechanical coupling investigated in skin tissue, as this is inherent to a 3D matrix. The use of 3D hydrogel systems to culture and study cell behavior allows better modeling of the in vivo conditions, permitting to tune the mechanical and morphological properties of the environment, and consequently studying cell response to different stimuli.
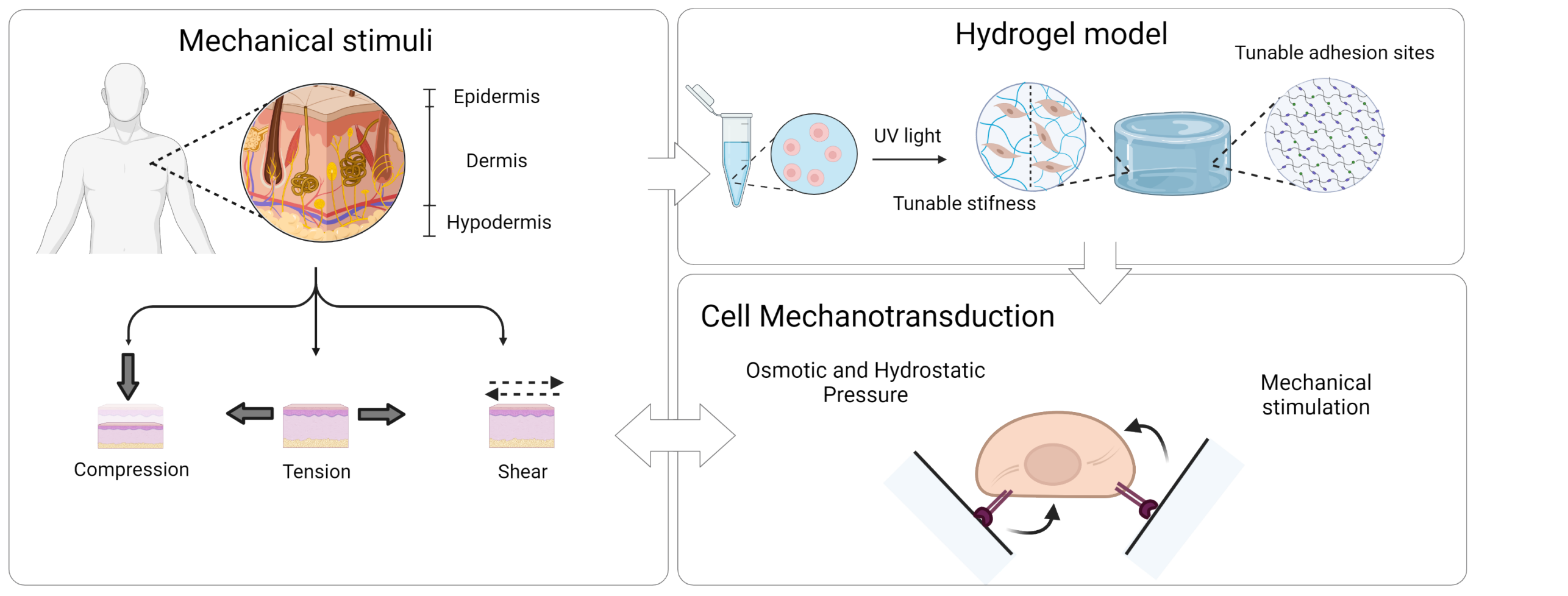
In our work, we use a 3D hydrogel systems with tunable stiffness and number of cell adhesion sites for human dermal fibroblast encapsulation, which we hypothesize can be used as a 3D model of the dermal niche. We aim to apply different mechanical and chemical stimuli to cells encapsulated within this platform and evaluate the cell biological response. At first we aim to characterize how the hydrogel system responds to the applied stimulation from a physical and mechanical point of view to evaluate how the stimulus is influenced by the synthetic 3D matrix, and hence how this is transferred to the cells. In the second part of the project, we will study the biological response of human dermal fibroblasts to dimensionality (2D vs 3D) and chemomechanical evaluating if these can induce fibroblast towards a pro proliferative or pro fibrotic state. Lastly we aim at better understanding which pathways are involved in the cell biological response envisioning to use this knowledge for future clinical and therapeutical applications.
Project Lead
Lorenza Garau Paganella
Collaborators
Macromolecular Engineering Lab, D-MAVT, ETH
Werner Group, Institute for Molecular Health Sciences, D-BIOL, ETH
Funding
This work is conducted as part of the SKINTEGRITY project (SKINTEGRITY.CH).
[1] R. A. Brown, R. Prajapati, D. A. McGrouther, I. V. Yannas, and M. Eastwood, "Tensional homeostasis in dermal fibroblasts: Mechanical responses to mechanical loading in three-dimensional substrates," J. Cell. Physiol., vol. 175, no. 3, pp. 323–332, 1998, doi: 10.1002/(SICI)1097-4652(199806)175:3<323::AID-JCP10>3.0.CO;2-6.
[2] J. H. C. Wang, B. P. Thampatty, J. S. Lin, and H. J. Im, "Mechanoregulation of gene expression in fibroblasts," Gene, vol. 391, no. 1–2, pp. 1–15, 2007, doi: 10.1016/j.gene.2007.01.014.
[3] A. Wahlsten et al., "Mechanical stimulation induces rapid fibroblast proliferation and accelerates the early maturation of human skin substitutes," Biomaterials, vol. 273, no. July 2020, p. 120779, 2021, doi: 10.1016/j.biomaterials.2021.120779.
[4] A. E. Ehret, K. Bircher, A. Stracuzzi, V. Marina, M. Zündel, and E. Mazza, "Inverse poroelasticity as a fundamental mechanism in biomechanics and mechanobiology," Nat. Commun., vol. 8, no. 1, 2017, doi: 10.1038/s41467-017-00801-3.
[5] K. Maki et al., "Hydrostatic pressure prevents chondrocyte differentiation through heterochromatin remodeling," J. Cell Sci., vol. 134, no. 2, 2021, doi: 10.1242/jcs.247643.
[6] K. Seltmann et al., "Humidity-regulated CLCA2 protects the epidermis from hyperosmotic stress," Sci. Transl. Med., vol. 10, no. 440, 2018, doi: 10.1126/scitranslmed.aao4650.
[7] H. Wang, M. W. Tibbitt, S. J. Langer, L. A. Leinwand, and K. S. Anseth, "Hydrogels preserve native phenotypes of valvular fi broblasts through an elasticity-regulated PI3K / AKT pathway," vol. 110, no. 48, 2013, doi: 10.1073/pnas.1306369110/-/DCSupplemental.www.pnas.org/cgi/doi/10.1073/pnas.1306369110.