Tissue aspiration
The tissue aspiration test is performed by establishing thorough contact between a probe head and a tissue, and creating a prescribed time-variable vacuum in the aspiration tube such that the tissue is sucked in through the aspiration hole. A camera captures the full side view profile of the deforming tissue. The first experimental set-up of this type at our institute was designed more than 20 years ago by Vuskovic and Kauer [1,2] with significant improvements for in-vivo applications introduced later [3,4]. In order to perform intraoperative measurements on humans, the measurement procedure must be non-traumatic, operate under sterile conditions and comply with the time- and space-limitations in the operating room, while at the same time allowing to maintain the appropriate control over the mechanical boundary conditions. During a typical experiment, a (negative) suction pressure is applied such that the displacement of the tissue apex is in the order of a few millimeters, and pressure data and images are recorded. Tissue from the surface down to about 10 mm depth contributes to the measured mechanical response.

The probe was designed such that it can be completely disassembled for cleaning, and for ethylene oxide gas or plasma sterilization. Time histories of measured pressure and deformation profiles are the input data for determining the biomechanical characteristics of the tissue investigated, either by extracting characteristic scalar parameters from the measured data [5-7], or by solving the inverse problem through numerical methods, i.e. by iterative comparisons between simulated and measured tissue response [8,9].
Based on the operation principle of the “standard” version of our device, used for example for in vivo measurements on human liver during abdominal surgery [3,4,9-12], dedicated and optimized devices have been developed for other medical applications, e.g. the measurements on the human cervix during pregnancy [5-7, 13-15], for prolapse diagnosis [16], for application on internal organs during laparoscopic interventions [4,17], and the characterization of facial tissues [18].
As an advantage of the aspiration technology, it can be useful as a diagnostic tool even without the complexity of solving the inverse problem. In fact, an assessment of the tissue state can be based on simple scalar quantities directly extracted from the experimental data such as parameters called “stiffness”, “creep”, “softening” or “rising time” introduced, e.g., for the classification of the response of the uterine cervix, liver or human skin [3-5,10,13,19-23].
The latest developments focus on a device for the in vivo mechanical testing of skin. The measurement principle of the “NIMBLE” is based on the standard version of the above-described devices and operates with a negative pressure to deform the tissue. In order to reduce operator effects the device’ size and weight was significantly reduced by implementing a displacement-controlled measurement mechanism [20]. With the “NIMBLE” we introduce a novel ultra-light suction device, that will support clinicians and skin experts with an easy-to-use tool for early-detection of microstructural changes in the skin tissue [21], caused by diseases, as well as longitudinal monitoring of disease progression [22,23].
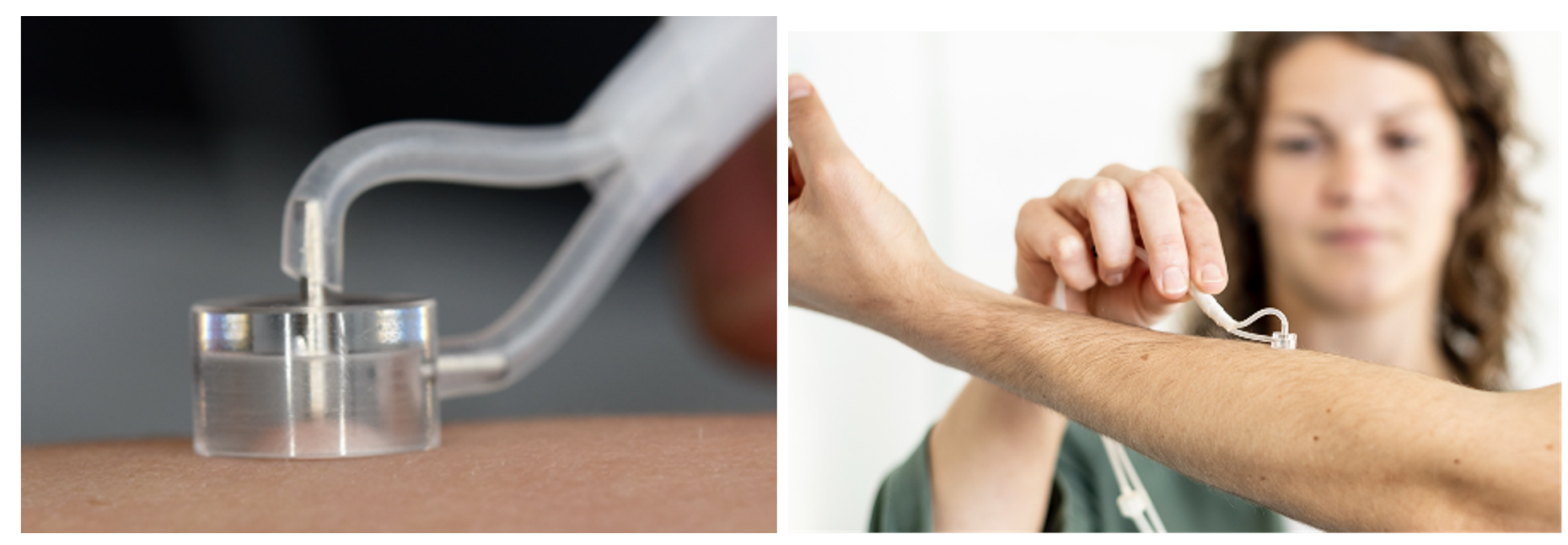
We aim at a commercialization of the NIMBLE as a medical device to improve skin care and enable doctors to more precisely diagnose skin diseases. This project is supported with a ETH Pioneer Fellowship.
[1] Vuscovic V. Device for in-vivo measurement of mechanical properties of internal human soft tissues. ETH Diss. Nr. 14222 (2001).
[2] Kauer M. Inverse finite element characterization of soft tissues with aspiration experiments. ETH Diss. Nr. 14233 (2001).
[3] Nava A. In vivo characterization of the mechanical response of soft human tissue, ETH Diss. Nr. 17060 (2007).
[4] Hollenstein M. Mechanics of the Human Liver: Experiments and Modeling, ETH Diss. Nr. 19587 (2011).
[5] Mazza E., Nava A., Bauer M., Winter R., Bajka M., Holzapfel G. A. (2006), Mechanical properties of the human uterine cervix: an in vivo study, Med. Image Anal. 10, 125-136. DOI: 10.1016/j.media.2005.06.001
[6] Bauer M., Mazza E., Nava A., Zeck W., Eder M., Bajka M., Cacho F., Lang U., Holzapfel G. A. (2007) In Vivo Characterization of the Mechanics of Human Uterine Cervices, Ann. NY Acad. Sci. 1101, Reproductive Biomechanics, 186-202. DOI: 10.1196/annals.1389.004
[7] Bauer M., Mazza E., Jabareen M., Sultan L., Bajka M., Lang U., Zimmermann R., Holzapfel G. A. (2009) Assessment of the in vivo biomechanical properties of the human uterine cervix in pregnancy using the aspiration test, Eur. J. Obstet. Gyn. R. B 144, 1, 77-81. DOI: 10.1016/j.ejogrb.2009.02.025
[8] Hollenstein M., Nava A., Valtorta D., Snedeker J. G., Mazza E. (2006) Mechanical Characterization of the Liver Capsule and Parenchyma, ISBMS 2006, Lect. Notes Comput. Sci. 4072, 150-158.
DOI: 10.1007/11790273_17
[9] Mazza E., Grau P., Hollenstein M., Bajka M. (2008) Constitutive modeling of human liver based on in vivo measurements, MICCAI 2008, Lect. Notes Comput. Sci. 5242, 726-733. DOI: 10.1007/978-3-540-85990-1_87
[10] Mazza E., Nava A., Hahnloser D., Jochum W., Bajka M., 2007, The mechanical response of human liver and its relation to histology: An in vivo study, Med. Image Anal. 11, 663-672. DOI: 10.1016/j.media.2007.06.010
[11] Nava A., Mazza E., Furrer M., Villiger P., Reinhart W. H. (2008) In vivo mechanical characterization of human liver, Med. Image Anal. 12, 203-216. DOI: 10.1016/j.media.2007.10.001
[12] Hollenstein M., Mazza E. (2012) Mechanical Characterization of the Human Liver, Eds: Holzapfel G., Kuhl E., Computer Models in Biomechanics: From Nano to Macro, Springer. DOI: 10.1007/978-94-007-5464-5_26
[13] Badir S., Bajka M., Mazza E. (2013) A novel procedure for the mechanical characterization of the uterine cervix during pregnancy. J. Mech. Behav. Biomed. Mater. 27, 143-153. DOI: 10.1016/j.jmbbm.2012.11.020
[14] Badir S., Mazza E., Zimmermann R. and Bajka M. (2013) Cervical softening occurs early in pregnancy: characterization of cervical stiffness in 100 healthy women using the aspiration technique. Prenat. Diagn., 3, 737-741. DOI: 10.1002/pd.4116
[15] Badir S. Towards a diagnostic device - mechanical characterization of the uterine cervix in pregnancy. ETH Diss. Nr. 22418 (2015).
[16] Röhrnbauer B., Betschart C., Perucchini D., Bajka M. Fink D., Maake C., Mazza E., Scheiner D.A. (2017) Measuring tissue displacement of the anterior vaginal wall using the novel aspiration technique in vivo. Sci. Rep. 7, 16141. DOI: 10.1038/s41598-017-16083-0
[17] Hollenstein M., Bugnard G., Joos R., Kropf S., Villiger P. Mazza E. (2013) Towards laparoscopic tissue aspiration, Med. Image Anal. 17, 1037-1045. DOI: 10.1016/j.media.2013.06.001
[18] Weickenmeier J. Jabareen M., Mazza E. (2015) Suction based mechanical characterization of superficial facial soft tissues. J. Biomech. 48, 4279-4286. DOI: 10.1016/j.jbiomech.2015.10.039
[19] Pensalfini M., Weickenmeier J., Rominger M., Santoprete R., Distler O., Mazza E. (2018) Location-specific mechanical response and morphology of facial soft tissues. J. Mech. Behav. Biomed. Mater 78, 108-115. DOI: 10.1016/j.jmbbm.2017.10.021
[20] Mueller B., Elrod J., Distler O., Schiestl C., Mazza E. On the Reliability of Suction Measurements for Skin Characterization (2020) J. Biomech. Eng. 143, 021002. DOI: 10.1115/1.4047661
[21] Müller B., Ruby L., Jordan S., Rominger M.B., Mazza E., Distler O. (2020) Validation of the suction device Nimble for the assessment of skin fibrosis in systemic sclerosis. Arthritis Res. Ther., 22, 128. DOI: 10.1186/s13075-020-02214-y
[22] Elrod J., Müller B., Mohr C., Meuli M., Mazza E., Schiestl C. (2019) An effective procedure for skin stiffness measurement to improve paediatric burn care. Burns 45, 1102-1111. DOI: 10.1016/j.burns.2019.02.004
[23] Müller B., Mazza E., Schiestl C., Elrod J. (2021) Longitudinal monitoring and prediction of long-term outcome of scar stiffness on pediatric patients. Burns Trauma 9, tkab028. DOI: 10.1093/burnst/tkab028