Research Topics
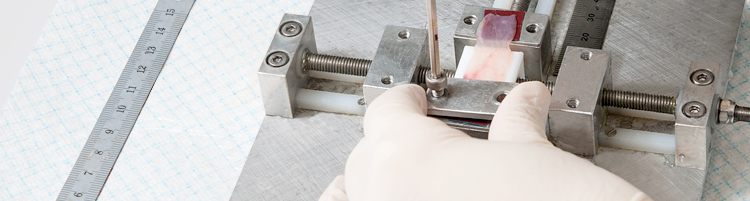
The group investigates the mechanics of biological and biomedical materials, i.e. man-made materials designed to interact with the body tissues in medical devices, implants, or scaffolds for tissue engineering. We study these material systems from sub-cellular to organ level towards a better understanding of complex interactions at different length scales. One important aspect is the evaluation of the so called “mechanical biocompatibility” of implants and prosthetics.
Our activities currently focus on the following topics:
Biological membranes and thin planar tissues represent mechanical structures with important physiological functions. Examples are the fetal membranes, the tympanic membrane, the thin capsules of abdominal organs, but also the thin layers of the skin. To understand these functions or possible dysfunctions and their relation to mechanical loads, we study the multiscale deformation behavior in homogeneous strain states as well as in the near-field of notches or cracks. Heterogeneous strain states are also investigated, for example related to the deformation behavior of excisional skin wounds and its changes during the healing process.
Associated Ongoing Projects:
- The Role of Chemomechanical Coupling in Skin and its Clinical Implications
- Skin Multilayer Mechanobiology
- Fracture Behavior of Elastomers and Soft Collagenous Tissues
- The Effect of Environmental Conditions on the Biomechanical Properties of Soft Collagenous Tissues
- A Computational Model of the Cytoskeletal Network of Endothelial Cell Layers
- Multiscale Characterization of Skin
The interaction between cells and their mechanical environment has become an emerging research topic due to the influence of e.g. substrate topography and stiffness, or marco- vs. microscopic deformability on cell behavior, such as their proliferation, differentiation, migration, and orientation. Providing cells in tissue-engineered materials and biomedical devices with an appropriate mechanical environment is essential for their successful integration in clinical applications; examples are the development of endothelialized cardiovascular devices within the Zurich Heart project or tissue-engineered skin substitutes in the Skintegrity project. We work with traction force microscopy for assessing cellular forces exerted on soft materials. We design dedicated dynamic bioreactors that provide controllable mechanical stimuli to cells. These tools are used to advance our understanding of the coupling between cell biology and mechanics.
Associated Ongoing Projects:
- Micro Topography Patterning of General Elastomer Surfaces for the Endothelialization of Ventricular Assist Devices
- Skin Multilayer Mechanobiology
- Conductive Skin
- A Computational Model of the Cytoskeletal Network of Endothelial Cell Layers
- The Effect of Hydrostatic Pressure on Endothelial Cells
- Endothelial Monolayer Response to Stretch and the Role of Senescence
- Development of a 3D Model of the Dermal Niche
- Characterization and Modelling of Biomaterials for Applications in Biohybrid Robotics
For materials used in contact with soft biological tissues and cells, there are particular requirements on the deformation behavior. Ideally, these materials match the mechanical properties of native tissues at all relevant length scales, a characteristic that we refer to as "mechanical biocompatibility". We investigate, for example, polymers, filamentous meshes for hernia repair, and hydrogels and electrospun scaffolds for tissue engineering. In addition to purely mechanical characteristics, e.g., deformation, stress, and stiffness, our work also considers coupled phenomena, such as the chemoelastic response of implant and scaffold materials , which might play an important role in transducing mechanical signals and establishing suitable mechanobiological environments for resident cells.
Associated Ongoing Projects:
An improved understanding of the mechanical behavior of native tissues and biomedical materials and the ability to perform computer-based simulations and mechanical analysis represent valuable resources for the development of biomedical equipment for therapy or diagnosis. In close collaboration with medical doctors and biologists, we work on simulations to better understand the interaction of these devices with the human body, and devise improved solutions. Another aspect concerns transferring tissue characterization techniques from in-vitro to in-vivo applications, e.g. as diagnostic methods to detect changes related physiological or pathological processes in the human body.