Tensile testing
Planar uniaxial and biaxial tensile tests play an important role in material characterization, and belong to the most widely applied experiments to analyze the deformation and fracture behavior of soft materials and tissues. It is of particular interest for incompressible-isotropic materials such as rubber, where planar testing can provide a complete characterization of the material properties, and for thin biological tissues, where these tests suffice to determine their membrane properties.
Our custom-made setup for planar-biaxial materials testing was specifically designed for soft materials and biological tissues. Up to four hydraulic actuators can be used, that are mounted on a positioning plate installed on a vibration isolation table. The primary configuration aligns the actuators in 90° angles for planar biaxial testing. The actuators can be driven individually in displacement or force control-mode, whereas the loading profile can be defined by the operator. The hydraulic system operating at 200 bar pressure allows testing at a wide range of loading rates up to 200 Hz under precise control of the loading profiles [1-4], and by use of different load cells we can measure forces from millinewtons to kilonewtons.
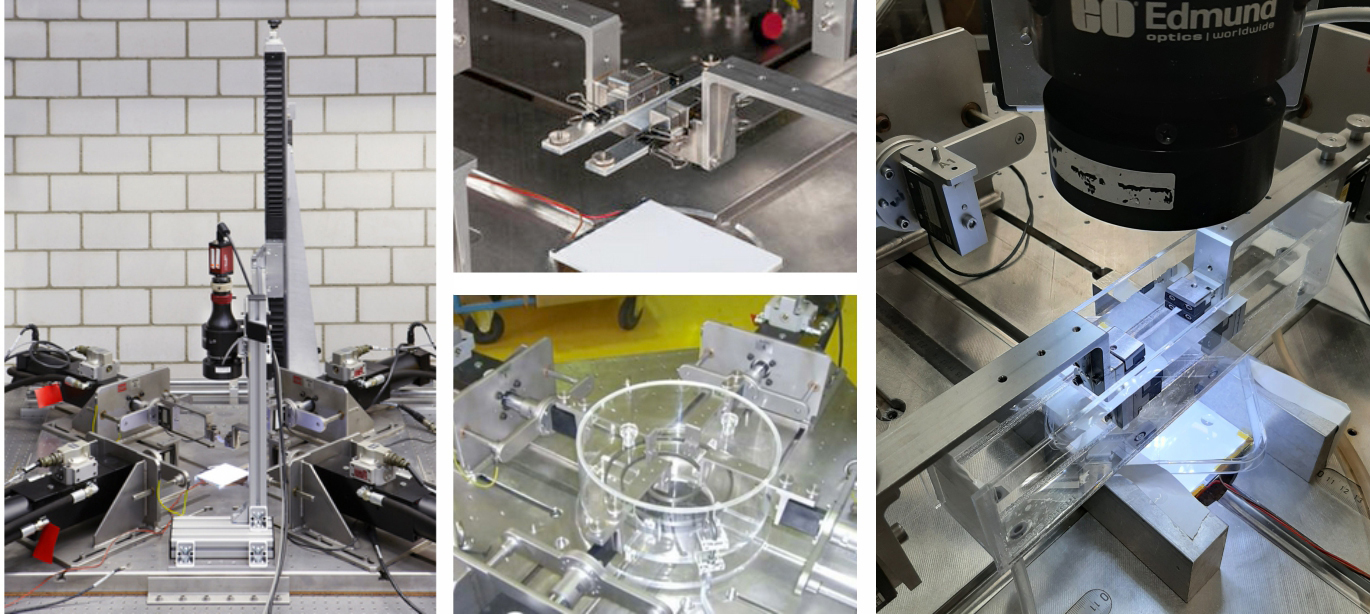
A biochamber can be installed for testing of materials and biological tissues in liquid environments at constant temperature, e.g. in a buffered saline bath at 37°C or in solutions of controlled osmolarity [5-8]. The testing system is equipped with a CCD camera system connected to a controller to record images of the deforming specimen surface at defined frame rates. We use different telecentric lenses to focus on the desired field of view and custom lighting solutions to optimize the visibility of the specimens. The image sequences are analyzed by custom software and scripts for digital image analysis. These tools allow tracking characteristic features at the sample surface, to determine the displacement field and to provide computational solutions of the deformations field.
While tensile testing belongs to the standard procedures in materials characterization, our methods are dedicated to the particularities of delicate soft biological tissues and very soft materials. For example, their high compliance complicates the definition of a stress-free reference state, so that "tare loads" need to be defined with care, or the reference state needs to be defined from the kinematics observed from the recorded images [9-11]. Moreover the typically pronounced strain-stiffening at higher loads requires special clamping designs and mounting techniques that guarantee secure fixation of the specimens without inducing damage.
We combine our tensile tests with constitutive model development, numerical simulation, histology [9] and other methods to analyze the microscopic anatomy of tissues in order to interpret and rationalize the observed mechanical properties.
[1] Ehret A.E., Hollenstein M., Mazza E., Itskov M., 2011, Porcine dermis in uniaxial cyclic loading: sample preparation, experimental results and modeling, J. Mech. Mater. Struct. 6, 1125-1136. DOI: 10.2140/jomms.2011.6.1125
[2] Hollenstein M., Ehret A.E., Itskov M., Mazza E. (2011) A novel experimental procedure based on pure shear testing of dermatome-cut samples applied to porcine skin. Biomech. Model. Mechanobiol. 10, 651-661. DOI: 10.1007/s10237-010-0263-1
[3] Mauri A., Perrini, M., Ehret, A.E., De Focatiis, D.S.A., & Mazza, E. (2015) Time-dependent mechanical behavior of human amnion: macroscopic and microscopic characterization. Acta Biomater.11, 314-323. DOI: 10.1016/j.actbio.2014.09.012
[4] Bircher K., Ehret A.E., Mazza E. (2016) Mechanical characteristics of bovine Glisson's Capsule as a model tissue for soft collagenous membranes. J. Biomech. Eng. 138, 081005. DOI: 10.1115/1.4033917
[5] Buerzle W., Mazza E. (2013) On the deformation behavior of human amnion. J. Biomech. 46, 1777-1783. DOI: 10.1016/j.jbiomech.2013.05.018
[6] Bernardi L., Hopf R., Ferrari A., Ehret A.E., Mazza E. (2016) On the large strain deformation behavior of silicone-based elastomers for biomedical applications. Polym. Test. 58, 189-198. DOI: 10.1016/j.polymertesting.2016.12.029.
[7] Ehret A.E., Bircher K., Stracuzzi A., Marina V., Zundel M., Mazza E. (2017) Inverse poroelasticity as a fundamental mechanism in biomechanics and mechanobiology. Nat. Commun. 8, 1002. DOI: 10.1038/s41467-017-00801-3
[8] Bircher K., Merluzzi R., Wahlsten A., Spiess D., Simões-Wüst A.P., Ochsenbein-Kölble N., Zimmermann R., Deprest J. Mazza, E. (2020) Influence of osmolarity and hydration on the tear resistance of the human amniotic membrane. J Biomech 98, 109419. DOI: 10.1016/j.jbiomech.2019.109419
[9] Hopf R. Modeling of highly deformable structures and materials for biomedical applications. ETH Diss. Nr. 23569 (2016)
[10] Hopf R., Bernardi L., Menze J., Zündel M., Mazza E., Ehret A.E. (2016) Experimental and theoretical analyses of the age-dependent large-strain behavior of Sylgard 184 (10:1) silicone elastomer. J. Mech. Behav. Biomed. Mater. 60, 425-437. DOI: 10.1016/j.jmbbm.2016.02.022
[11] Domaschke S., Mechanical multiscale modelling of fibrous materials with application to electrospun networks. ETH Diss. Nr. 26328 (2019).
[12] Jabareen M., Mallik A.S., Bilic G., Zisch A.H., Mazza E. (2009) Relation between mechanical properties and microstructure of human fetal membranes: an attempt towards a quantitative analysis. Eur J. Obst. Gyn. Rep. Biol. 144, S134-S141. DOI: 10.1016/j.ejogrb.2009.02.032